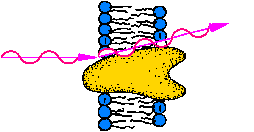 |
Membrane Pharmacy Structure Dynamics
Research group : Priv.Doz. Dr.
Thomas Nawroth
Molecular
Motion
in proteins and motile polymers
|
Topics : Induced fit, Catalytic
domain motion, Structural regulation,
Motile
polymers
Protein structure and dynamics
Proteins bearing catalytic functions, i.e. enzymes, especially those of
cellular
energy metabolism, motor proteins, key proteins of cell regulation
and receptors are capable of molecular motion. This feature depicts the
fact that a variety of proteins exist in more than one structure (fold).
A structural flexibility is facilitated in membrane
proteins by the hydrophobic effect (binding without localized forces,
entropy-driven).
Induced fit
The flexibility of proteins is generally a consequence of the composition
of proteins from one ore more long chains of polypeptides, which
form a structure in space by folding. Between the fold elements, helices,
beta-sheets and loops, mostly no covalent chemical bonds but weak interactions
are present (hydrogen bridges, ionic pairs, hydrophobic interactions etc.).
Thus most proteins are a rather "soft matter", which show some structural
flexibilty when strong interactions to other molecules occur. This general
small structural rearrangement inside enzymes is depicted by the "induced
fit" model of substrate-enzyme interaction: In many cases the resting form
of an enzyme fits not perfectly the structure of its substrate. The perfect
molecular fit occurs only after substrate to protein binding by a structural
rearrangement of the protein "wrapping arround the substrate". Nevertheless
also in cases where no induced fit occurs small oscillations and motions
of the protein structure contribute to the biological function, e.g. side
group rotation of amino acids.
Catalytic domain motion
A second class of. larger strutural rearrangements is the displacement
of whole structure domains, e.g. protein subunits, during a catalytic function
or work. In this case the rearranged domain may be rather large, e.g. having
>10,000 mass. The displacment distance is orders higher as in the induced
fit case, e.g. 1 nm or more. The displacement is of transient nature, which
means that it occurs only for a short time during catalysis. Then the protein
is rearranged to its resting structure. Those catalytic domain motions
are typical for bioenergetic proteins (energy converters) and molecular
motors, e.g. muscle. In both cases the protein can store energy inside
the rearranged structure. Those transient molecular motions can only be
detected by time resolved methods, e.g. time resolved small angle scattering
(TR-SAXS). A prrequsite is the synchronization of a macroscopic sample
by a jump method, e.g. by sudden increase of the substrate concentration
by rapid mixing with a stopped flow device or by photochemical activation
of a burried form from caged-compounds or temperature jump. A succesful
example is the estimation of transient structural
changes in working F1ATPase during the reaction cycle of ATP
hydrolysis.
Structural regulation
The structural regulation is a common principle of regulation processes
in cells in the millisecond to minute time scale. At longer time (> 30
min) the regulation occors at the genetic level, i.e. by activation or
down regulation of genes and subsequently the amount of special proteins
beeing present in the cells. The structural regulation depicts a molecular
regulation of proteins (enzymes) by switching over their structure from
one stable fold to another, which differ in their biological activity.
Enzymea having that feature are depicted as allosteric proteins. The structural
switch can be triggered by reversible (loose) binding of an effector molecule
or by chemical modification, e.g. phosphorylation, or proteolytic cleavage.
Those regulative proteins are oftenly located at the end or beginning of
a catalytic chain (regulative key function). Well known examples are the
activation of cell metabolism by G-protein coupled hormone receptors recognizing
external siganls, e.g. Adrenaline, or light in case of the visual system,
and the structural regulation of ATP-synthase
and its catalytic head, the F1ATPase.
Motile polymers
Polymers may consist of similar chemical components as proteins, but show
mostly a lower degree of molecular order. This is a consequence of the
actual techniques of polymerization, which can be improved, e.g. by topochemical
polymerization or sequence specific block-condensation. Polymers of molecular
order show improved properties, e.g. Kevlar (TM). Some polymers are sensitive
to environment factors, e.g. temperature, light or pH. Thus the combination
of technology and knowledge of proteins and polymers appears to be possible
in the coming decade. The promising result would be polymers capable of
active motion, i.e."motile polymers". Nevertheless before that two problems
have to be solved: i) the energy input into the polymer system has to be
established, for example by light as in the biological photosynthesis,
or by electrical power, and ii) the analytic detection of molecular motion
has to be developed in the nanosecond to millisecond range, which is the
time scale of domain motions (the smaller, the faster). This requires the
development of regioselective structure labeling techniques and the construction
and long term funding of pulsed radiation sources for neutrons and X-ray
/ Synchrotron light, e.g. the pulsed free electron laser FEL.
email to: nawroth@MPSD.de
update : 11.10.2013