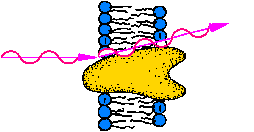 |
Membrane Pharmacy Structure Dynamics
Research group : Priv.Doz. Dr.
Thomas Nawroth
Neutrons
and neutron scattering
|
Contents
General
Neutron scattering
Neutron sources
Neutron instruments
Neutron detectors
General
Neutrons are a powerful tool for the structure investigation of macromolecules,
e.g proteins, membranes, polymers and complex materials. In contrast to
X-ray photons they are scattered by the atomic nucleus. The scattering
power varies widely by the isotope. The energy of the radiation is usually
in the milli-eV range (cold neutrons), whereas X-ray photons exhibit keV
energy. As a consequence neutrons may also be used for inelastic and quasielastic
scattering, which allows the detection of energy transfer processes and
energy levels in a sample. Generally neutrons are required in structural
biology and polymer science for:
1. structure estimation of non-crystalline matter at high contrast,
e.g. membranes from protonated lipids in D2O-buffer.
2. distinguishing components of molecular complexes by their
contrast respective to the scattering power, which is called contrast variation.
3. detection of molecular motion in molecular complexes by time
resolved neutron scattering, e.g. of liposomes with incorporated membrane
protein
4. structure investigation of sensitive macromolecular solutions
with negligible radiation damage (cold neutrons).
5. structure investigation of very large macromolecules and complexes
(nanoparticles, large membrane structures).
6. detection of energy levels and transfer by inelastic or quasielestic
scattering, which is measured by "time of flight" (TOF) experiments with
a pulsed beam.
7. In material science the magnetic scattering is of wide importance
(domains, grains, spin glasses).
Neutron scattering
The neutrons passing an atom are scattered in a magnetic process by spin-spin
interaction. The scattering process can quantitatively be described by
the Quantum Chromo Dynamics theory, QCD. Thus the scattering is sensitive
to magnetic structures and spin polarization. The scattering probability
per absorbed particle is about a factor of 100 (H) to 1000 (D) higher,
as compared to X-ray photons (1:100,000). If no activalable metals (Cd,
Gd etc.) are present in the sample, there is no radiation damage with cold
neutrons (8 Angstroem wavelength = 20 K level). This and the high contrast
of protonated material versus D2O makes neutron scattering powerful for
structure investigation of biological and polymer samples.
Because of the spin dependence, the scattering power of hydrogen and
deuterium varies by more than one order for neutrons. Thus with a biological
sample the neutron beam is scattered mainly by the hydrogen entity, in
contrast to X-rays, which see the electron density (mainly carbon, oxygen,
nitrogen ...). This offers a splendid opportunity of distinguishing
between the components of a macromolecular complex: The scattering intensity
depends on the contrast, i.e. the scattering length difference between
solvent and particle. This may be choosen by varying the deuterium content
in the solvent, i.e. H2O/D2O exchange, or in some case by partial deuteration
of the sample, e.g. by growth of bacteria in D2O and isolation of the deuterated
constituents. A similar effect can be achieved by magnetic spin polarization.
If the solvent exhibits the same scattering length density (power) as one
component of a complex, the scattering amplitude of this becomes zero,
i.e. it is matched. Thus the component structure may be investigated by
contrast variation in situ.
The contrast of protonated biological membranes and amphiphilic polymers
in D2O-buffer solution is much higher, as compared to X-rays, where the
contrast of lipid membranes in H2O is nearly zero. As a consequence lipid
membranes (liposomes) yield at the most powerful neutron scattering beamline,
the D22 instrument at the ILL, Grenoble,
an intenser signal than at the best high flux synchrotrons, while the neutron
small angle scattering (SANS) of a protein solution at this beamline is
equivalent to X-ray small angle scattering (SAXS) at a flux of 1011
ph/s, i.e. 1% of the flux of the most powerful monochromatic X-ray beamline,
the ID2A instrument at the ESRF, Grenoble.
This and the lack of radiation damage with cold neutrons explains the superior
role of neutron scattering in membrane structure research.
Neutron sources
Neutrons can be produced by three techniques:
- Neutrons can be produced by nuclear reactions, e.g. in a Radium/Beryllium
source. The maximal flux of this technology of <1011 n/s.cm2
is too low for the structure investigation of biological specimen or polymers.
- Neutrons can be produced by nucelar fission in a conventional reactor,
e.g. of 235U (1-2 excess neutrons / fission). The flux
in a commerial power plant reactor of 1012 - 1013
n/s.cm2 is to small for most scientific applications. Thus special
high flux reactors have been constructed, which supply a flux of 1014
- 1015 n/s.cm2 , which is required especially for
the weak scattering biological specimen and polymers. The most powerful
neutron source for science is the Institut
Laue Langevin,
ILL at Grenoble. Most
applications use a permanent monochromatic neutron beam, while a pulsed
beam is produced by a chopper-absorber (1 - 10% beam yield) for quasielastic
or inelastic experiments (TOF).
Properties of some european neutron
sources
Source
|
Flux (with intruments), power
|
Organization
|
Examples of scientific use
|
ILL-HFR :
ILL-Grenoble
|
1015 n/s.cm2 , 58 MW
|
european contract
|
proteins, membranes, polymers, time-resolved scattering, TOF |
FRJ-2
: IFF-FZ Jülich
|
1.5 x 1014 n/s.cm2 , 24 MW
|
national
|
polymers, membranes, material science reflectiometr. |
FRM-II : TUM Munich-Garching
(under construction)
|
5 x 1014 n/s.cm2
|
national + european
|
proteins, membranes, polymers, time-resolved-scattering, TOF |
PSI , Villingen
SINQ spallation neutron source
|
equivalent to 1014 n/s.cm2
|
national + european
|
polymers, membranes, material science
|
- Neutrons can be produced by a novel technique in a spallation source,
which may yield a pulsed peak flux of 1018 n/s.cm2
and an avearge flux of 1015 n/s.cm2. This requires
no fission reactor but a high flux high energy proton accelerator. The
proton beam of typically 1000 GeV energy hits a heavy metal target. Because
the absorbed energy is much higher than the nuclear binding energy, the
intermediate nuclei are spread into many small fragments of short livetime
and up to 100 neutrons / incoming proton. If a linear accelerator with
(optional) bunch compressor synchrotron is used, the proton and neutron
beams are pulsed, which is optimal for TOF and kinetic studies. Additional
to the neutrons, an intense meson beam is produced, which may be used for
medical applications (cancer). The European community plans to build a
large european spallation source, the ESS. Small pulsed spallation sources
are located in the United Kingdom and in Japon; Switzerland has reconstructued
a cyclotron for a spallation source SINQ
(non pulsed) recently.
Neutron instruments
Neutron detectors
In contrast to X-rays, neutrons cannot be detected directly with a gas
detector or a CCD camera, because of the lack of an electric chage. Thus
the neutron signal has to be converted to a secondary signal prior to detection,
e.g. by a nuclear reaction, which produces a gamma photon, or by a scintillator,
which is a light emitter.
- The signal of a scintillator, e.g. a Lithium glass, can be measured
by a set of photomultiplier tubes (Anger camera). The spatial resolution
and flux is limited by technical reasons. A further problem is the limited
lifetime of the photomultiplier tubes.
- The most commonly used neutron detectors are gas detectors with BF3
or 3He as medium. The secondary gamma photon is measured as usual for X-rays
(gas amplification, Geiger-Mueller principle). Many neutron scattering
instruments have 2D gas-detectors of about 1 cm spatial resolution. The
signal is picked up by a XY frame grid and converted by delay line and
clock devices to a digital adress of the hit at the detector suface. A
problem for time resolved measurements and of strong scattering specimen
is the count rate limitation of the current neutron detectors (< 200,000
/s). Thus improved detectors for experiments at high flux sources are under
development at the ILL.
email to: nawroth@MPSD.de
update : 11.02.2014